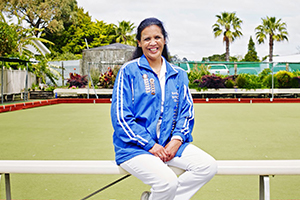
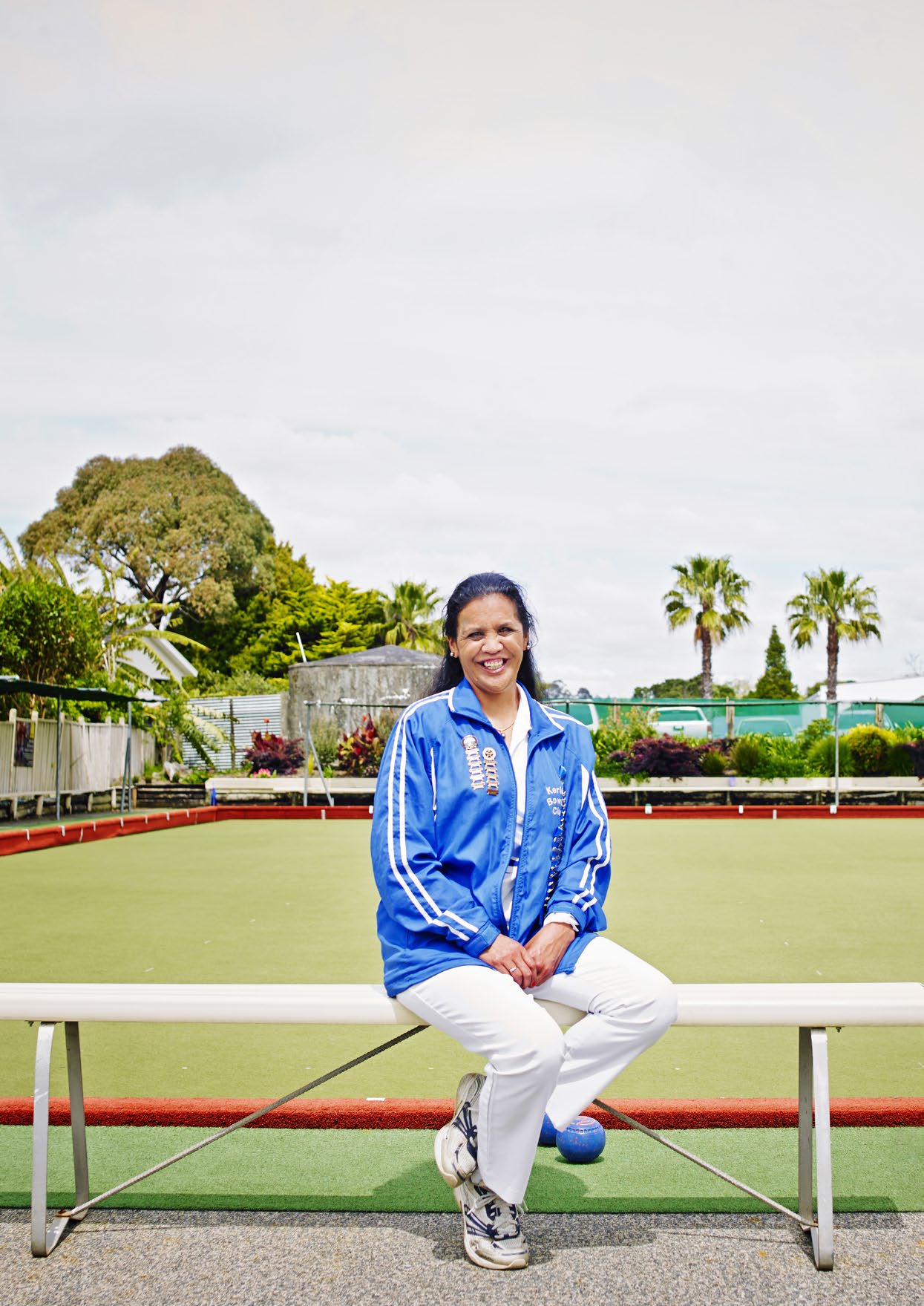
A tiny piece of her brain could save yours
At the Centre for Brain Research, patients give Auckland scientists a rare and invaluable advantage in the global search for treatments for Alzheimer’s, autism, epilepsy and other neurological conditions.
By Donna Chisholm
Left – Three decades after her surgery, Becky Papera is thriving. Now 41, she lives in Paihia and plays outdoor bowls competitively.
Photo : Victoria Birkinshaw
The seizures were destroying Becky Papera’s life. They started when she was just 18 months old, in the early 1980s. After the kids at her Northland school found out about her epilepsy, the bullying was relentless. She dropped out when she was 15 because she couldn’t follow the lessons anymore. But the seizures meant she couldn’t get a job, either. At 19, she was given the chance to undergo brain surgery that could cure her, or leave her little better — or at worst, kill her.
Papera’s parents, a laboratory charge technologist and a nurse, believed the risk was too great and counselled her against it. But Papera had had enough: “I thought, ‘If it works, it works. If I die, I die. Either way, I won’t get teased anymore.’”
In June 1999, at Auckland Hospital, neurosurgeon Edward Mee drilled into her brain just above her left ear and removed a small section of her hippocampus, where nerve cells had died and caused scarring — the likely source of her seizures.
In most hospitals, this tissue is discarded after a pathologist examines it and makes a diagnosis. But at Auckland Hospital, since the late 1980s, it has been collected and taken across the road to a team of researchers working with Professor Richard Faull, the renowned neuroscientist. Faull realised it was a precious resource that could help them understand the causes of brain diseases that afflict tens of thousands of New Zealanders.
Papera not only survived the surgery — she thrived. She hasn’t had a single epileptic seizure since. And the tiny section of her tissue that ended up in Faull’s laboratory has played its part in changing the direction of brain research in New Zealand.
One of the scientists who studied Papera’s tissue was molecular pharmacologist and neuroscientist Mike Dragunow, who still works closely with Faull — now Sir Richard Faull — at Auckland University’s Centre for Brain Research (CBR). Dragunow would look for neurochemical changes in the tissue that might explain the cause of seizures — something that is still not well understood. “The type of work we did with Becky’s tissue, and that of other wonderful donors, has really led to everything else we do,” says Dragunow.
Twenty-one years after Papera’s surgery, the scientists are growing human brain cells themselves and using them to test possible treatments. No other laboratory in the world does this in the same way. There are research centres with bigger brain banks. But they usually lack the rich clinical and family background information that CBR holds on the tissue of its donors. Researchers collate this through fostering close relationships with patients, their families and clinicians, in advance of the donation.
I thought, ‘if it works, it works. If I die. Either way, I won’t get teased any more.’
In 2019, CBR marked its 10th anniversary. That year, Papera, who lives in Paihia, came to visit the centre. Her only wish for her visit was to thank Mee for giving back her life, and the scientists for the lives they will save in the future. Instead, she found Faull, Dragunow and Mee lining up to thank her. “It’s about collaborative research between brain scientists, doctors and families,” says Faull. “This is probably one of the best examples ever. A person has had an operation which has changed her life and her tissue will be used to change other lives.”
Despite the CBR’s groundbreaking work, a couple of years ago, Auckland University had to cut the centre’s funding, forcing Faull into a desperate scramble for sponsors. It is now heavily supported by private donations, including a $16.5 million gift from the Hugh Green Foundation — the single biggest gift the university has ever received. The money will support the Hugh Green Biobank in perpetuity and endow a professor of translational neuroscience to take the work of the scientists’ work from the laboratory to the people. “The families have the greatest knowledge of all because they are living with the challenges of brain disease 24/7.”
The Hugh Green Biobank, led by Professor Dragunow, is the facility in which human brain cells are grown and studied. Along with the Neurological Foundation Human Brain Bank — the CBR’s repository of tissue from 700 donated brains and biopsies from more than 300 neurosurgical operations at Auckland City Hospital — it has enabled the centre to develop leading-edge science.
In 2015, it became the first research group in the world to grow human brain cells, which is arguably the most important of all its work. The rest of the world’s focus remains on animal models of brain disease for drug development, which, as clinical trials demonstrate, often lead to major stumbling blocks when therapies are tried in humans.
If the avuncular Faull is the famous face of the centre, Dragunow, who began working with Faull as a post-doctoral fellow 30 years ago, is head gardener of its brain-cell nursery. Get him talking about the cells he’s growing — pericytes, microglia and astrocytes — and his conversation fires up like an overexcited synapse as he explains how clever they are. Today, the pericytes and microglia are scrapping it out for favouritism as he brings up colourful images of the pair on the computer screen on his paper-free desk.
The pericytes control the blood-brain barrier, keeping toxins and other cells out. In patients with Alzheimer’s disease, stroke and head injury, that barrier breaks down. Dragunow and his team are growing pericytes from patients with different brain diseases, and then testing drugs on them to see if they can shore up the barrier by improving the health of the cells.
If the centre can develop drugs that do this, it would be an exciting advance. Inflammation of the pericytes might be one of the causes of blood-brain barrier leakage, and also trigger inflammation in other brain cells, says Dragunow.
Then there’s the microglia. If they had a job description it would be “garbage collector”, because they’re the cells that effectively empty your brain cells of trash. Microglia are vital for the brain’s immune response to infection, trauma or reduced oxygen supply. If they sense a bacterial infection, for example, they can eat the bacteria like a waste disposal unit. The problem with microglia, though, is that they are sometimes less than discerning — are they in fact disposing of things they shouldn’t, such as neurons? So research which initially investigated improving the function of the microglia to take out more trash has in some ways been turned on its head.
Dragunow says there’s also growing interest in the role of the microglia in conditions such as epilepsy. “We know there is significant inflammation in the brain of people with epilepsy and some of these inflammatory cells are the microglia. There’s a wee bit of thought around the world now that what inflammatory cells release can actually cause seizures. So when we study the microglia, it’s about learning to control their activity with drugs. It’s really exciting and it all built on the work we started with the sort of tissue Becky and our other donors gave us.”
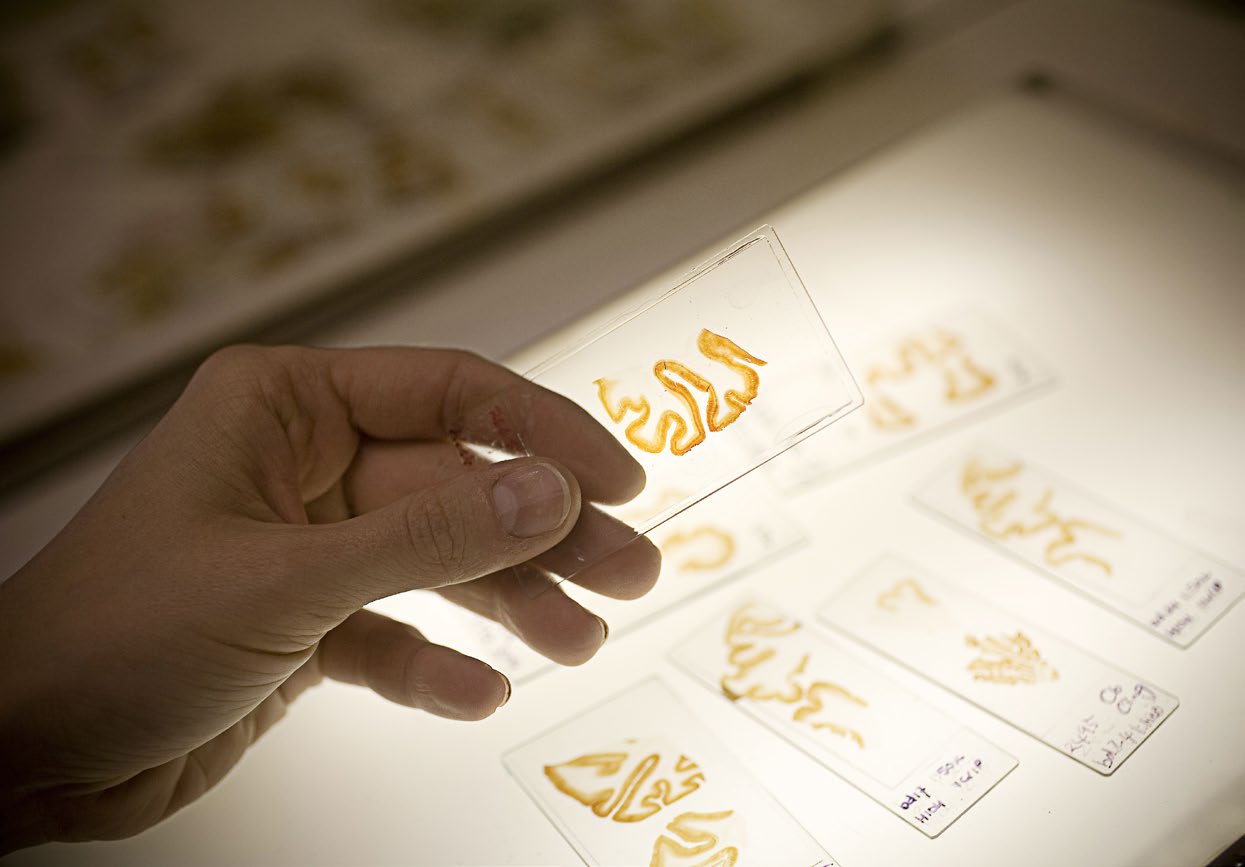
Slides of non-human stained brain tissue under analysis. Photo: Adrian Malloch.
Every day, scientists at the Centre for Brain Research turn skin cells into brain cells. Professor Bronwen Connor, who heads the centre’s Neural Programming and Repair Laboratory, says the Nobel Prize-winning discovery by English and Japanese researchers in 2007 is still “very sci-fi in my mind”.
Revolutionary is a word often overused these days, but it fits the finding that mature cells can be genetically reprogrammed to become stem cells, which are capable of developing into any tissue of the body. The Auckland scientists have added their own twist to the process. They didn’t want to produce stem cells, which can also develop into cancer-inducing tumour cells. Instead, Connor’s team added two genes, SOX2 and PAX6, to adult human skin cells to create what they call a brain precursor cell. SOX2 and PAX6 are the first two genes that get turned on in the embryo when the neural tube — which becomes the baby’s spinal cord, skull and brain — is being formed. Over a period of two or three weeks, the precursor brain cell develops into a mature brain cell.
In recent years, the researchers have added different chemical cues to make different sorts of brain cells — the dopamine-producing neurons which, when lost, cause Parkinson’s disease; the GABA neurons that are lost in Huntington’s disease; and the cortical neurons affected in autism.
And here’s where the work becomes truly breathtaking. Connor’s team has found that the skin-derived reprogrammed brain cells survive and mature after being transplanted into the brains of rats with Huntington’s or Parkinson’s. If transplanted cells can restore motor and sensory function in the rats, Connor says, scientists could potentially use the patients’ own skin to generate replacement brain cells to treat their conditions.
The beauty of cell reprogramming, Connor explains, is the ability to take the skin cells from patients who have the diseases, particularly if those conditions are inherited. “Do they grow differently? Do they express different genes? Do some genes turn on earlier or later? Do some not come in at all?”
Connor says they’ve found that mature GABA brain cells grown from the skin of Huntington’s patients look different to those grown from patients without the disease. In the late stages of maturation, the cells have much smaller bodies and fewer neurites — the “arms” sticking out from the cell body. “A healthy neuron looks like a tree, with branches and sub-branches. The Huntington’s ones have smaller bodies and maybe just a couple of branches.”
The brain cells that the centre produces are now being used to screen possible drug therapies for both Huntington’s disease and Fragile X syndrome, a genetic condition that causes learning and cognitive problems in children. The cortical brain cells grown from the skin of Fragile X patients also look different at maturity — they have long, ineffective neurites that don’t make proper connections. Connor hopes the centre can develop a treatment that could be given to babies postnatally, while the brain is still developing, that would give them a better quality of life.
“All of our drugs to date have been developed in rodents, and then have gone into clinical trial in patients,” says Connor. “Only about 10 per cent of the drugs that go into clinical trial get FDA approval and the main reason they fail is efficacy. They’ve gone into lab rodents, which are inbred and genetically similar, and then they hit a human population where everyone’s genetics are different. What this allows us to do is have little human patients in a dish.”
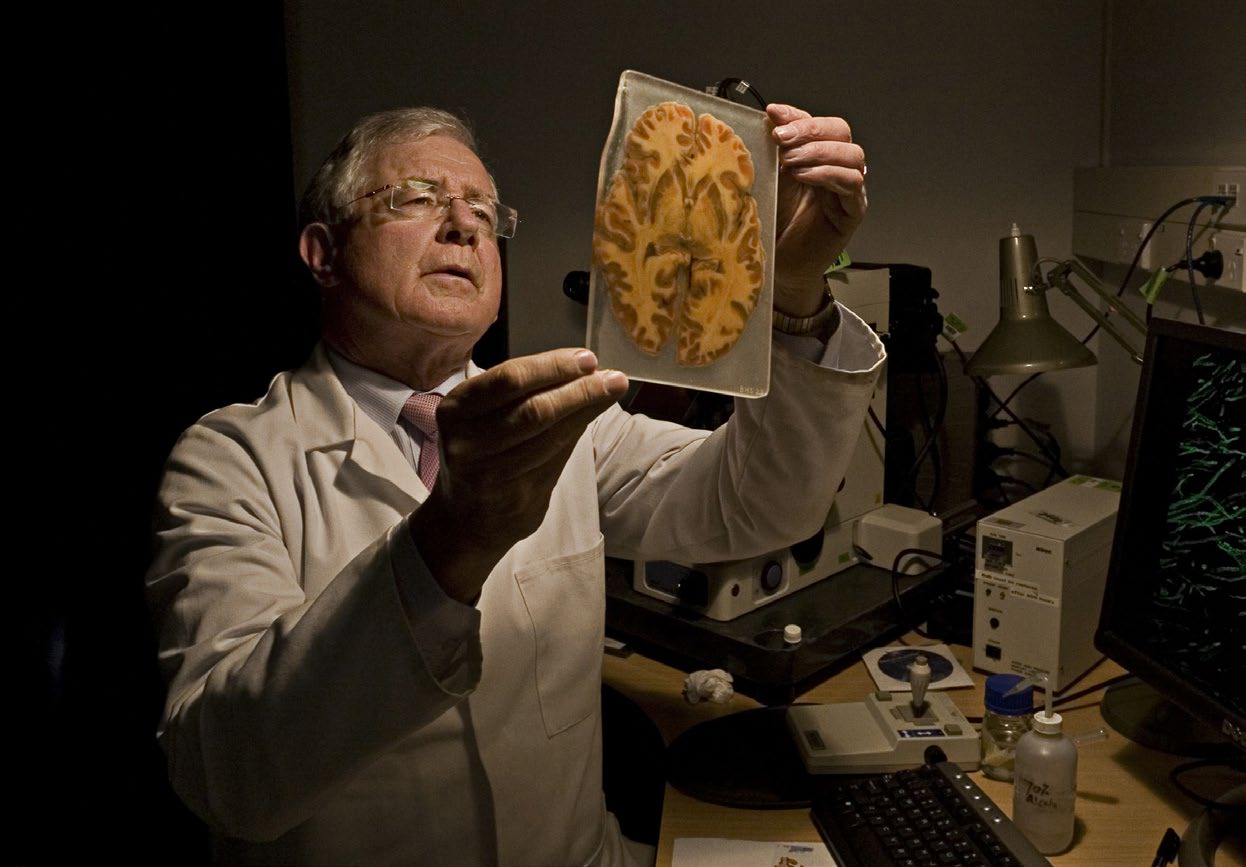
Neuroscientist Sir Richard Faull realised that brain tissue from surgical patients could be used to understand neurological conditions affecting tens of thousands of New Zealanders. Photo: Adrian Malloch.
One of the many mysteries of Alzheimers’ disease, until recently, was why people with the condition also have a high incidence of gum disease. Scientists didn’t know whether gum bacteria was a potential cause of Alzheimer’s, or stemmed from poor dental hygiene as a result of the disease.
Then, in January 2019, Californian scientists linked the gum disease gingivitis to the development of Alzheimer’s. The finding became one of the year’s most discussed scientific papers around the world. Dr Steve Dominy, the chief scientific officer of Cortexyme, the biotech start-up that sponsored the research, says this crucial connection could not have been made without CBR, and its research comparing 50 Alzheimer’s and non-Alzheimer’s brains. “Without their work, we wouldn’t have been able to publish the paper.”
The Californian study confirmed that the gingivalis bacterium was present in the brains of people with Alzheimer’s, not just their mouths. The more toxins the bacteria produce in a person’s brain, the more extensive Alzheimer’s symptoms the researchers found. (Some sceptics have suggested that if the gingivalis bacterium is indeed to blame, our ancestors who lived without toothbrushes, floss or toothpaste, should have been more susceptible to Alzheimer’s. The reality is, of course, that they didn’t live long enough to develop it.)
Cortexyme has designed a drug that attacks the gingipain toxins. After passing an initial safety trial in healthy older people, it is now going to clinical trial in the United States.
Meanwhile, two other CBR scientists, Dr Jessie Jacobsen and Dr Emma Scotter, are attempting to understand the many genetic roadmaps that lead people to develop autism or motor neurone disease (MND). About 100 “high-confidence” genes have been identified — inherit just one and you’ll be autistic. There are around 600 to 800 others thought to play some role in making the condition more likely.
Jacobsen is part of a group led by geneticist Professor Russell Snell that has genetically tested some 500 people with autism, and several hundreds more of their non-autistic parents or siblings. “I’m learning that parents actually love just having an answer to why their child is the way they are,” she says. “I really underestimated how much value that had. It relieves the stress of thinking it was something they did or happened during conception.”
The work has thrown up between 20 and 50 new genetic variants that Jacobsen and Scotter believe are highly likely to cause autism. Snell emphasises that the work is not aimed at “doing away with” autism, but better understanding it.
And here’s where the work becomes truly breathtaking. Scientists could potentially use the patients’ own skin to generate replacement brain cells to treat their conditions.
Jacobsen is also working to develop a genetic screening tool for children with autism spectrum disorder. The work will include a large number of Māori and Pacific families, who she says have been under-represented in previous studies.
Another arm of the autism research is working on gut instinct — literally. Microbial ecologist Associate Professor Mike Taylor and Counties Manukau District Health Board developmental paediatrician Pratima Giri have been investigating links between gut health and autism. In a study that began early in 2019, they’ve recruited 100 children with autism or their neurotypical siblings with the aim of genetically sequencing the bacteria from their fecal samples. Results from about 50 samples show that the gut microbes of both are very similar. Taylor says this suggests that any influence of gut bacteria may be less related to what organisms are present, and more to do with what the organisms are actually doing. Giri’s team is also collecting data on the children’s diets, gastrointestinal issues such as constipation and diarrhoea, and other health characteristics.
Together with another CBR scientist, Taylor is also studying a mouse model of autism to investigate the effect of dietary micronutrients on autism-related behaviours and the microbiome — all the microbes in our gut. “There are so many different genes where mutations have been found that are associated with autism and it may well be the same for the microbiome. Everyone on the spectrum may be a bit different and individualised,” Taylor says. “It makes it very complicated to try to find patterns but also quite exciting if you think about opportunities for personalised therapy.”
Scotter’s group is genetically testing up to 300 patients with MND, which causes weakness and wasting in the limbs — British physicist Stephen Hawking died of the disease in 2018. Scientists have identified mutations in about 35 genes that can cause MND. However, those cases are in the minority: around 85 per cent of cases are “sporadic”, or without an identifiable cause. The disease affects men more commonly than women, and symptoms generally appear when people are in their 50s.
Scotter says there is a striking relationship between MND and a disease called frontotemporal lobe dementia — about half of MND patients have some features of this dementia and it’s thought that the same genes are involved in both conditions.
Much of Scotter’s work relies on tissue from MND patients held in the centre’s biobank. It’s examined for clumps of proteins, which are a common feature in many neurodegenerative conditions, including Alzheimer’s. Scotter says these are likely to be a symptom rather than a cause. The gene therapies being developed and tested are designed to turn down the mutant versions of the genes in the hope that fewer clumps form.
Much of the coverage of MND in New Zealand in recent years has been about the possible involvement of environmental toxins, for example the toxic gas methyl bromide, used at ports to fumigate imported logs and equipment. Reports highlighted a cluster of six MND cases among Nelson port workers between 1995 and 2005. A longitudinal study in Christchurch between 1985 and 2006 found the highest incidence of the disease in the world, with 2.2 deaths for every 100,000 people, although Australia isn’t far behind on 2.1.
Because no genetic studies were done on the Nelson group, Scotter says it is impossible to know whether there was simply a higher density of people in that area with a particular mutation. “People really want answers. They are trying to understand how you can get this terrible disease out of the blue. In some ways, maybe it’s helpful to have something to latch on to and feel they can rage against but it may draw attention away from the areas we need to focus on more. We need a thorough understanding of the genetic landscape of MND in New Zealand, whether we look the same as other countries and whether we have the same MND-causing genes.”
One of her priorities is to engage with Māori and Pacific populations to find out if there are any genes specific to those ethnicities. Māori seem to have lower rates of MND but it’s not known whether that is due to barriers to access in diagnostic tools.
Scotter says the longstanding relationships the scientists have with the families after tissue or brains are donated is vital. “I frequently go back to the clinicians and families and ask more questions and to tell them what we have found. It’s about the three pillars of the CBR — the brain researchers, the neurologists and neurosurgeons, and the families.”
Becky Papera is now 41 years old. She no longer lives in fear of seizures. She still has the genetic condition neurofibromatosis, which her epilepsy was part of and which can impair learning. As a result, she cannot work, but leads a very full life. She excels at outdoor bowls, has taken a course in fishing, and is confident and happy. “I’m just enjoying every single day,” she says. “Life is good.”
Her mother, Betty Papera, says her daughter had always been compassionate, friendly and outgoing as a teen. But after the operation, “her confidence just went through the roof”, she recalls. “She is whole again.” Betty and her husband Ina hope that publicity about their daughter’s brain-tissue donation will encourage others, particularly Māori and Pacific families, to more readily contribute their own.
“Unless we allow the research, education and technology to be done we will never gain all the knowledge,” says Betty. “Yes, it is a taonga, but the treasure needs to be understood and to be used, to be effective for future generations.”
Donna Chisholm is a North & South contributing writer.
This story appeared in the December 2020 issue of North & South.