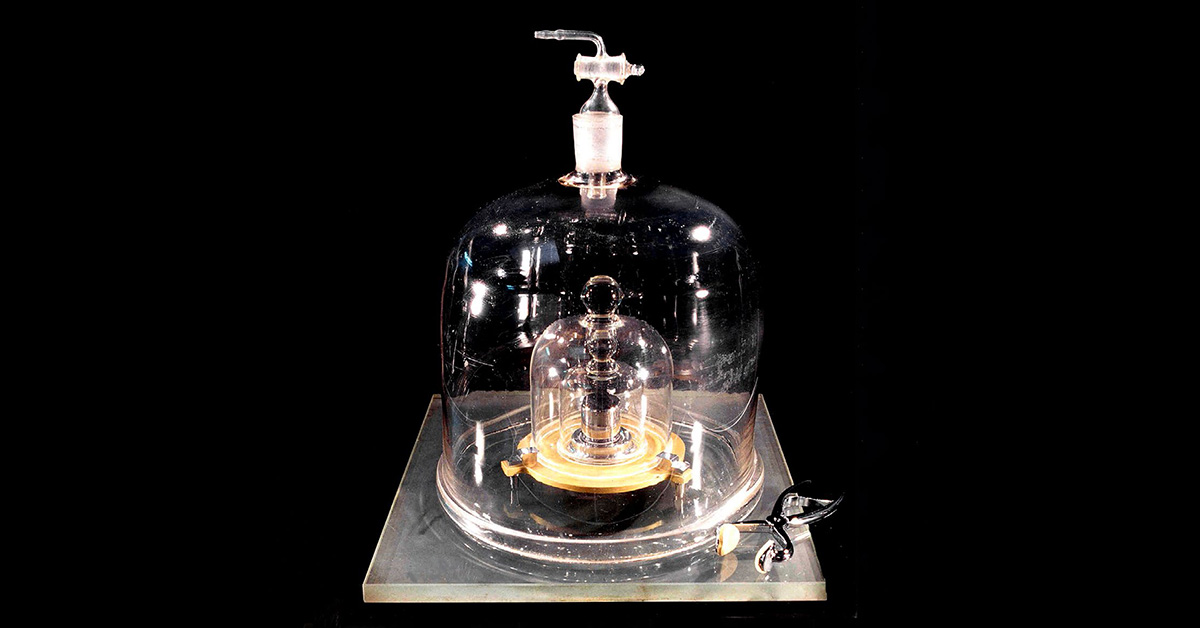
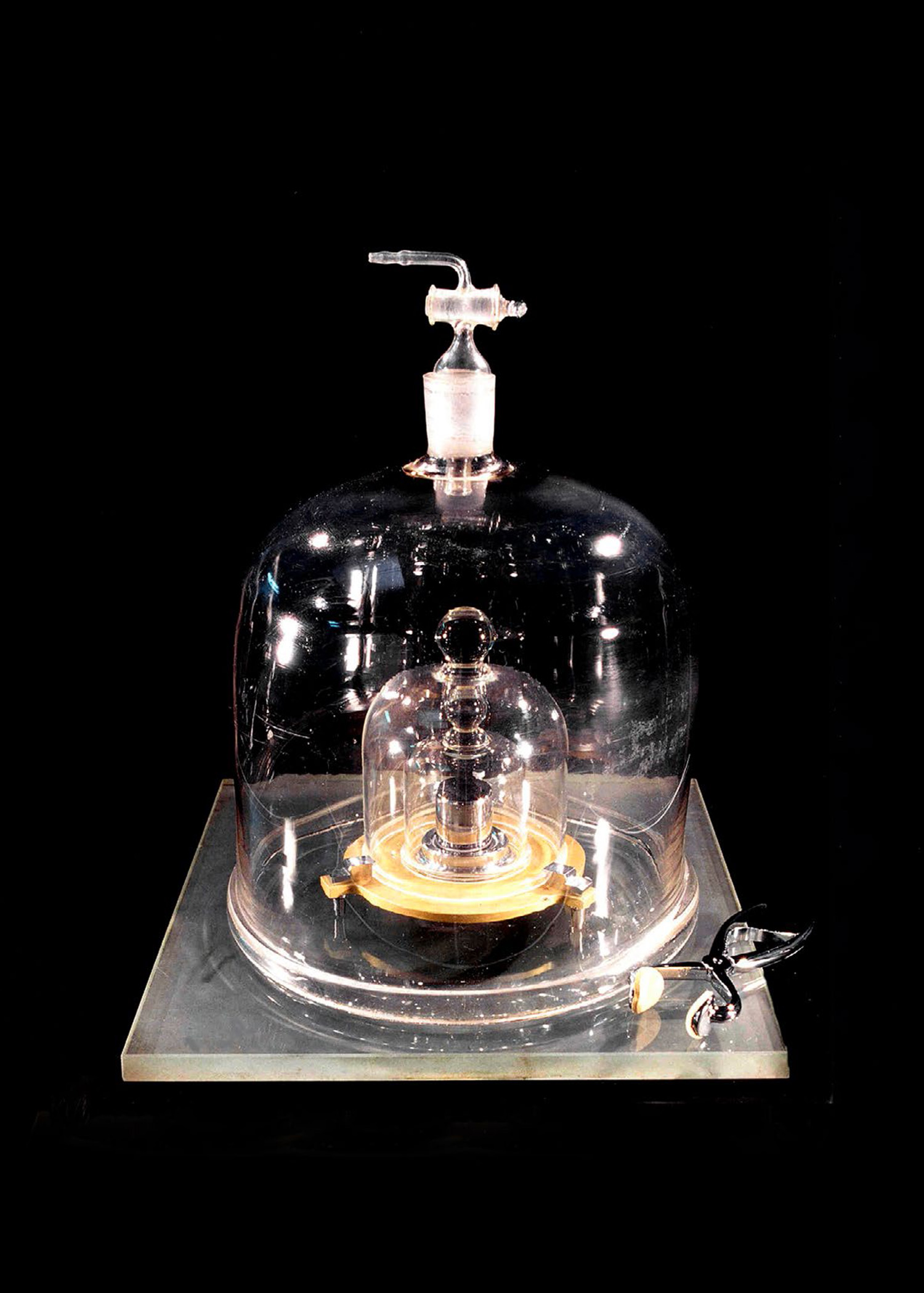
Weight of Expectation
How much is a kilogram? In a nondescript lab in Wellington, a team of metrologists is working on an instrument that will answer that question to a mind-scrambling level of accuracy.
By Mirjam Guesgen
For more than a century, the world of weight has relied on a single block of metal: Le Grand K, the literal grandmaster of kilograms. Photo: International Bureau of Weights and Measures, CC.
It’s time for the weekly shop, another Sunday chore to tick off. You navigate the aisles, grabbing what you need. In amongst the groceries in your trolley you’ve chucked a bag of flour; a bunch of bananas; a couple of nice cuts of meat. At the checkout, each little bleep of the self checkout machine brings up your price — $2.49 for one-and-a-half kilos of flour, $6 for your kilo of bananas and, ouch, $19.45 for the half kilo of steak.
You can trust that the weight the machine measured (and the price you paid) was right — that you don’t end up short-changed on your steak dinner — because the scales tell you. And the scales work because the people who made them calibrated them during production. But how did they know the kilogram they used for reference was accurate? How does anyone?
When it comes to time, every hour, minute and second is measured against the average from hundreds of clocks around the world, which create the references for the world’s time zones. When it comes to weight, on the other hand, the worldwide reference point was for more than 130 years one silvery block of metal that sits locked away, deep underground in Paris, in an environmentally controlled vault. Called Le Grand K, it’s literally the grand master of kilograms. Every few years, countries would ship their own reference blocks to Paris to be vetted against the master — including the one we still use here in New Zealand.
Every five years, this special “transfer” kilogram takes a trip to Paris and, after making its overseas voyage, returns home to the Measurement Standards Laboratory, New Zealand’s national metrology institute, tucked away at the base of the Wainuiomata hill in Wellington. Once metrologists (measurement scientists) know how much the transfer kilogram weighs, they use it to calibrate the three holy grails of New Zealand’s kilo. Known as “primary standards”, these three blocks are then used to calibrate “working standards”, which are what all of New Zealand’s trade weights are measured against, tuning up everything from supermarket scales to massive industrial weighers.
These primary standards will never leave the lab. Each is carefully stored inside a metal cupboard secured by an ornate key, sitting cloched under a glass bell — the room looks as much like an antiquities museum as it does a science lab. The hunks of metal are only handled very occasionally by trained staff, to avoid amateur handlers leaving tiny, atom-sized droplets of chemicals from dirt, sweat or even soap, which build up and make the kilo heavier over time. Hidden in wooden boxes nearby are other, smaller standards, roughly the size of AA or disk batteries that are used as the yardstick for weights like the milligram. Open the box to look at one of these and you have to hold your breath to avoid messing with their mass.
Everything in the lab must be handled with extreme care, the primary standards most of all. An accidental sneeze onto a working weight would be embarrassing, but could be fixed with a meticulous cleaning regime. Disrupt one of the primary standards, though, and you’re looking at an even more complex and laborious task — and you’d have messed with an even more crucial part of the standardisation process. Fixing a primary standard involves heating up a cleaning solution to the exact right temperature and letting its vapour condense onto the metal. It’s fiddly, tricky and time consuming — something you’d want to avoid doing too often.
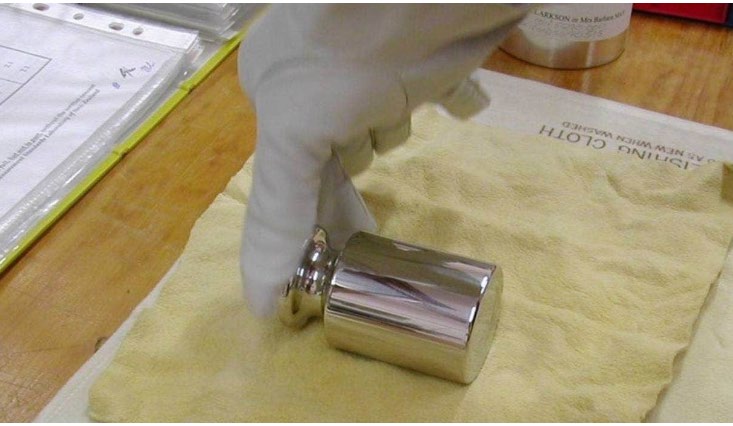
A metal transfer weight is carefully wrapped in a chamois before being flown to Paris for calibration. Photo courtesy Measurement Standards Laboratory of New Zealand (MSL).
The method of making and comparing blocks in order to standardise weight has been around since 1889, when Le Grand K was formed by order of the General Conference on Weights and Measures. Scientists and diplomats at the four-yearly conferences have the final say on any new decisions to do with measuring. They decide how much, how big, how long, how fast. The conference still exists today because of the Metre Convention — a treaty between more than 60 countries, including New Zealand, which decided all the weights and measures around the world should be the same, and that each country would promise to stick to it (despite its name, signatories of the treaty do not necessarily use the metric system domestically — the United States, for example, is a member state). Having global standards of measurement builds trust when it comes to trade: a kilo of milk powder here is the same as the kilo of milk powder that arrives in China after export.
But Le Grand K’s very singular grandness makes it vulnerable. Storing the standard in any way that’s less than perfect, handling it without the exact proper care and even just the passing of time slowly changes its weight, taking what constitutes a kilogram along with it. This means that when countries check their primary standards against Le Grand K (ours was last sent to France in September 2020), they can’t be sure it’s quite right, meaning the chance of discrepancies in weights between countries increases over time — and if Le Grand K ever went missing or was stolen or destroyed, the measurement world could fall into chaos.
There’s also the fact that the need for precise smaller measurements has grown, particularly as technological advances mean demand for ever-teensier parts for equipment such as phones and computers has increased. Scientists need something better than what we’ve currently got. There’s a limit to how small you can make a metal block; the smaller you go, the easier it is for a block’s weight to change based on something as seemingly inconsequential like breathing near it. Just like your hands have tiny molecules of sweat or dust, your breath contains tiny molecules of organic chemicals that land and stick to the metal.That means at a certain point, you can only calibrate machines based on parts of a whole — half a milligram, for instance, or a sixteenth of a gram — which causes the accuracy to begin to fall, making the measurement unreliable. This might not matter so much when it comes to buying fruit, but it matters a great deal when you’re developing a heart medication.
That’s where metrologists come in. For years they’ve been working away in the background, feverishly scribbling on blackboards to figure out how to tie all basic measurements — including mass, time and length — to something more stable than a block of metal or plank of wood (the “metre stick”). Instead of physical objects, it was decided at the General Conference on Weights and Measures in Versailles in 2018 that new definitions based on universal constants would be created: quantities that stay the same no matter what or where in the universe you are.
One well-known example of a universal constant is the speed of light, set at a steady 299,792,458 metres per second. Flip that around and you get the new definition of a metre — that is, how far a beam of light in a vacuum travels in 1/29979458-th of a second. Scientists can use instruments that are capable of measuring the time a light beam takes to travel a distance extremely accurately. This is called “realising the unit”.
The electromechanical measuring instrument used for realising weight is called the Kibble Balance. Since 2019 the kilo has officially been affixed to what’s called “Planck’s constant”, a fundamental and unchanging concept in quantum mechanics. In theory, this means Le Grand K is now obsolete — little more than a museum piece. In reality, the world is still in a period of transition. This is because Planck’s constant is only useful if you have a working Kibble Balance on hand to realise it. So far, only a handful of countries around the world have realised the kilogram using a Kibble Balance, including the United States, United Kingdom, Canada, France, Switzerland, China and South Korea. Their balances are enormous; sometimes the size of an entire room.
Our Measurement Standards Laboratory is one of fewer than a dozen labs anywhere taking on the challenge of building such a device. And, relative to their overseas counterparts at least, our metrologists are doing it on a shoestring budget. It’s a Number 8 Wire mentality, updated for the 21st century.
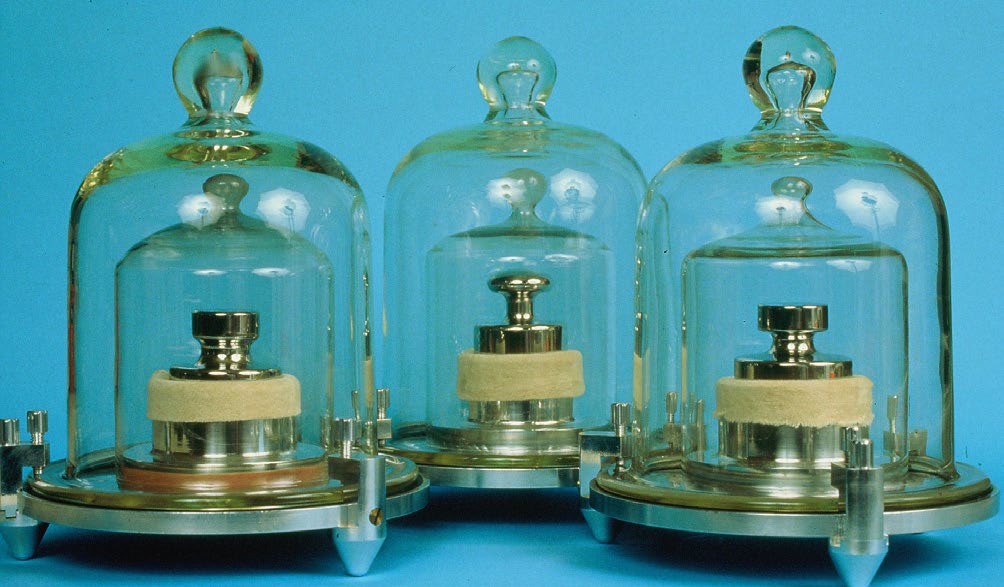
New Zealand’s three primary kilograms, below, in double bell jars which keep them free of weight-altering debris. Photo courtesy Measurement Standards Laboratory of New Zealand (MSL).
Yin Hsien Fung is in his mid-30s, a hiplooking guy with black, thick-framed glasses like an American indie musician. He’s one of the metrologists leading the Measurements Standard Laboratory’s project to make a kilogram-setting device.
Together with his colleagues Rebecca Hawke and Peter McDowall he takes me to their lab, down at the end of the main corridor of the building. The lab itself is about half the size of a standard school classroom and we shuffle cautiously to fit everyone inside, being careful not to disturb the array of equipment and parts neatly arranged around us.
Initially, I’m not exactly sure what I’m looking at, or why. There’s a trolley lined with towels storing a selection of different sized metal rings, numerous mystery boxes with wires running in and out of them, lasers and a winch mounted from the ceiling. Hawke pulls a delicate chain from a drawer and draws my attention over to what looks like a large cylindrical metallic drum, the kind professional brewers might use for making craft beer.
Standing an arm’s length away she holds the chain up. It reaches toward the drum, pulled in by an invisible force. “Don’t stand too close,” warns Fung. I imagine my metal retainer dragging me face-first towards the barrel. Donning a pair of leather gloves he slides a triangular wedge of metal off the top of the drum, revealing the powerful magnet inside.
This magnet and its casing, collectively called the “magnet system”, forms half of the Kibble Balance — the machine that should, when it’s finished, be able to accurately and reliably determine the kilogram in New Zealand using universal constants. Essentially, the Kibble Balance acts in a similar way to an old-school set of balance scales, but instead of balancing one kilogram weight against another, it pits a pulling, gravitational force against an electromagnetic force (the second half of the balance), which is created by an electric current inside a magnetic field. The New Zealand Kibble Balance uses a pressure sensor to measure how those two forces are balanced. Think of the pressure sensor as like looking at a spirit level to see if the two sides of the scale are even, except with much greater precision.
Building one of these balances is no simple task. Just to get to the point of building the magnet inside the barrel took close to five years of work. Getting the magnet to sit inside its metallic casing was a particularly tricky challenge. The gap between the magnet and the case is less than the thickness of a piece of paper, the magnets are fragile and brittle, and their magnetic force is pulling the casing from all directions with six tonnes of force. It took six people, a lot of creative thinking, and several days to lower the casing over the magnet without having it crash forcefully into one of the casing’s sides and break. “There wasn’t a lot of room for error,” McDowall says. “You only really get the one shot.”
Fung, Hawke and McDowall are the latest generation of scientists to tackle the challenge, guided by Mark Clarkson, a metrologist who’s been working in the field since 1990. In fact, Clarkson is a bit of a guru in the metrology community, with other metrology institutes worldwide looking to him for advice, training and consultancy. He was one of the first to start thinking about building a Kibble Balance here. “In those days, the only viable option seemed to be building something as big as the ones at the National Institute of Standards and Technology [in the US] or the National Physical Lab [in the UK], which was beyond our capability,” he says. To get super precise measurements, many other countries developing Kibble Balances are relying on massive magnets that cost a near-fortune and are truly enormous — some can reach up to a storey tall. Fung’s team is trying to make a unique, smaller version, with a fraction of the operating budget of their overseas counterparts. It’s like being at the dawn of computers, where everyone else was working with room-sized machines and you’re trying to jump straight to making a PC. “We’ve got a few complicated things to work through,” says Hawke. This seems like an understatement.
The next step is putting the magnet system (in fact, the whole Kibble Balance) inside a vacuum chamber. This will mean that the measurements will be done in a stable ambient condition, unaffected”by air. After the magnet is placed in the vacuum chamber, the team will build up the remaining parts from there. The magnet itself (the “heart” of the balance) only represents half of the device — one arm of the balancing scales if you will. The team still needs to build the part which will hold the weight to be measured and a high precision pressure sensor which tells when the mass is balanced.
This ampere balance, bellow, is shown at the US National Bureau of Standards (now known as the National Institute of Standards and Technology, or NIST) in 1927. It is something of a precursor to NIST’s Kibble balance which has been operating in the US since 2015.
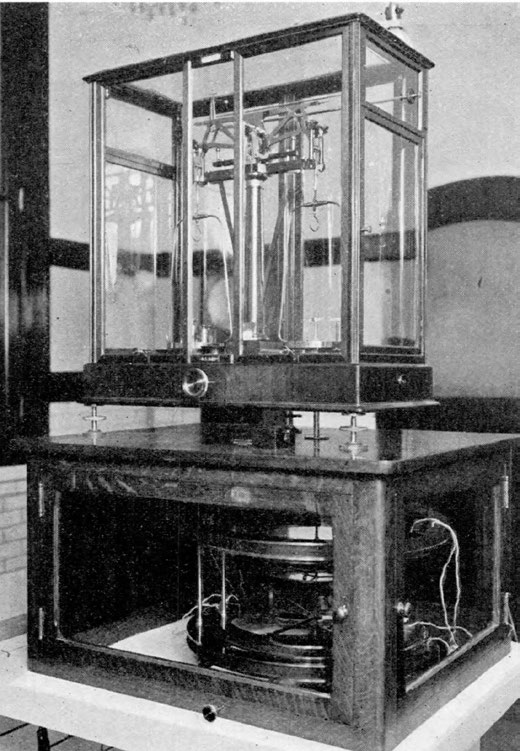
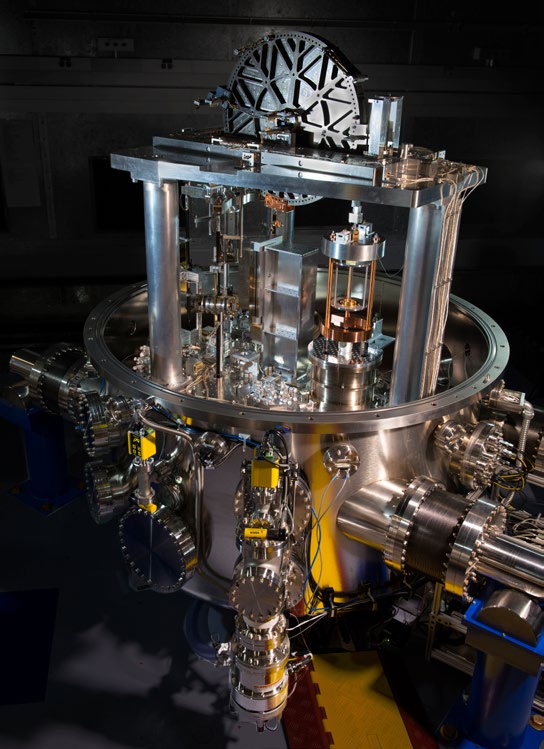
Photos courtesy Measurement Standards Laboratory of New Zealand (MSL).
They don’t just need to finish building the thing, they also need to build it so it’s accurate enough to count among the world’s other Kibble Balances. That means measuring every single part of the balance super accurately, from the voltage and current of the electricity circuit to how much gravitational force the kilogram is pulling with. All to an accuracy of 20 parts per billion — or within 0.0000002. “That will be our target to get us into the game,” says Fung. Right now, the equation they’re using consists of letters representing forces. The end goal is to replace some of those letters with numbers. Once they have it, the team can use the value as part of an equation to work out how much a kilogram weighs.
The Measurement Standards Laboratory is the only lab in the southern hemisphere to try this. The other labs involved are all in countries known as scientific and economic powerhouses. “We’re showing that New Zealand can do it,” Clarkson says. Fung agrees, adding that he hopes that by building a Kibble Balance here, New Zealand can retain its science talent, and even end up recruiting more budding scientists from overseas. “Maybe I’m too ambitious, who knows?”
As well as creating a more reliable weight standard, the Kibble Balance also represents a new frontier of measurement tools that could help scientists make new discoveries in the field of physics. (The ultimate applications of it are unfathomable at this stage.) A similar thing happened after the advent of the atomic clock, which keeps track of time by measuring the vibration of atoms. After atomic clocks were built, someone got the idea to mount them inside some of the Global Positioning System (GPS) satellites orbiting the Earth. Because GPS devices work by measuring how long it takes signals from different satellites around Earth to reach it, millisecond differences in time can translate to distances of hundreds of kilometres. Getting a more precise time measurement meant location estimates improved too, eventually resulting in the creation of things like the maps application you use on your phone. Certainly, no one at the start could have imagined that by building a better clock, we’d end up being able to navigate the world with a computer in our pocket.
Scientific endeavour aside, the Kibble Balance will serve a real, applied function. New Zealand has a legal obligation under the Measurement Standards Act 1992 (the law that keeps us in line with the Metre Convention) to set and measure units to global standards. Once finished, the balance will eventually have its own place at the Measurement Standards Laboratory, and it will become the go-to device for setting the kilogram here, in turn calibrating any machine in the country that relies on mass; from home scales to weighbridges.
Fung and his team plan to hang on to the metal primary standards locked away in the lab’s cupboards, though. They’re keen to measure them and see how much their weight has changed over the last decade, mostly out of curiosity. Besides, there’s a certain sentimentality that comes with metrology. “We have a way of hanging on to things,” says Fung, gesturing to other measurement artifacts and keepsakes which form a kind of mini-museum in their hallways, and which includes a smaller Lego version of the Kibble Balance their team built for fun.
Much like the metallic rods currently in careful storage in the lab, few people will see this incredible machine once it’s finished. Those needing something calibrated precisely, such as testing labs, government bodies, research institutes, manufacturing plants or pharmaceutical companies, will periodically send in their own kilogram weights to be checked by the highly accurate balance machine. The balance might tell them that their “kilos” actually weigh 0.999998 kg or 1.000002, allowing the companies to fine tune their measuring instruments accordingly.
This system will mean a more resilient, more stable and more precise kilogram. We’ll keep weighing out our bananas at the supermarket, keep stepping on the scales at home and keep taking our medication without a second thought. That’s the weird thing about metrology, says Fung. If they got things wrong, it would be all over the news: “We messed up New Zealand’s entire weight measurement system.” But, if they do their jobs well, hardly anyone will realise what they’ve achieved.
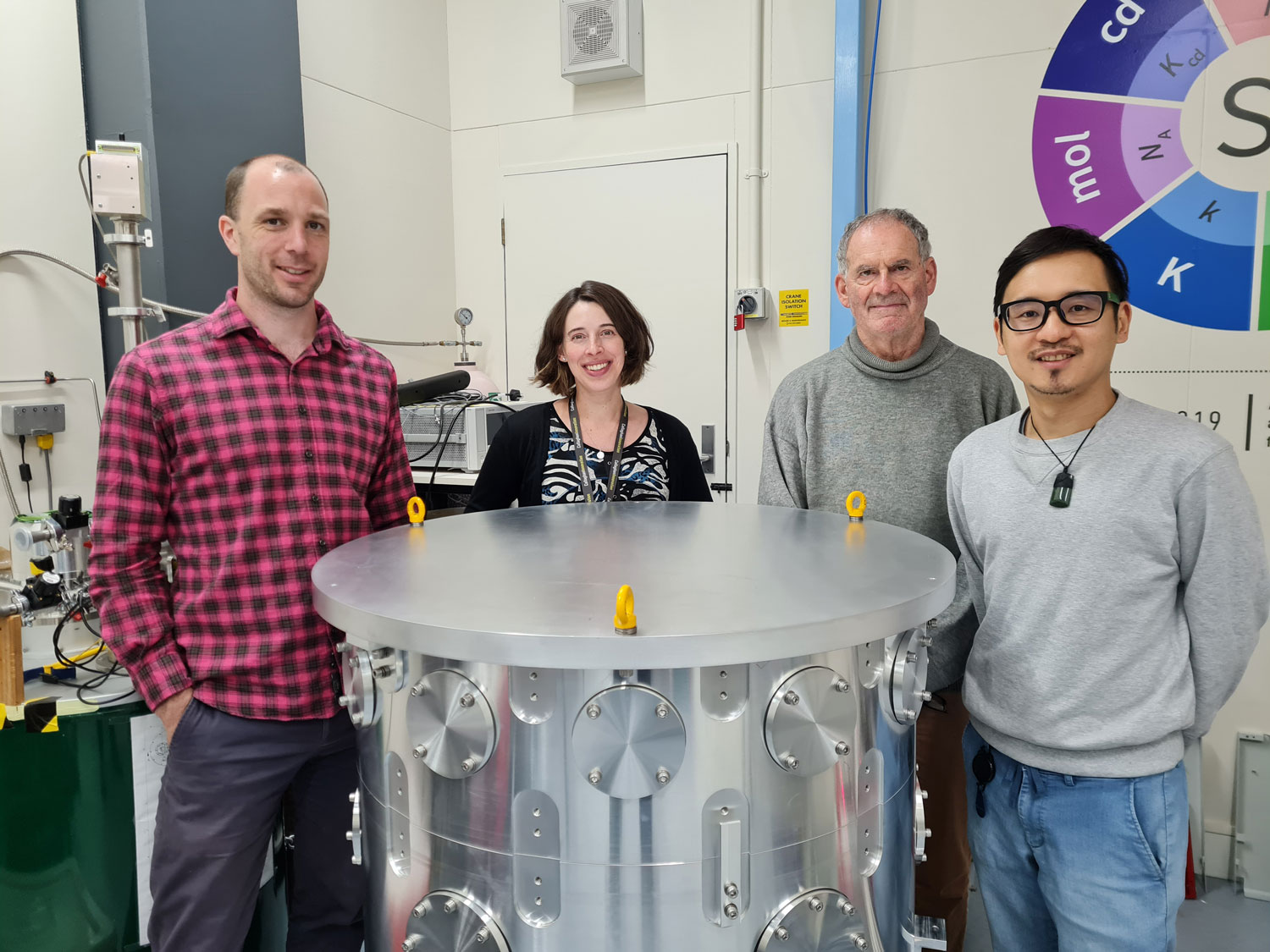
Peter McDowall, Rebecca Hawke, Mark Clarkson and Yin Hsien Fung, pictured from left to right, stand around MSL’s Kibble Balance vacuum chamber.
Mirjam Guesgen is a freelance science journalist. Formerly in Wellington, she now lives in Canada.
This story appeared in the December 2022 issue of North & South.